Hardware Development of Sample Shuttling in High-Field NMR
Dr. Chingyu Chou
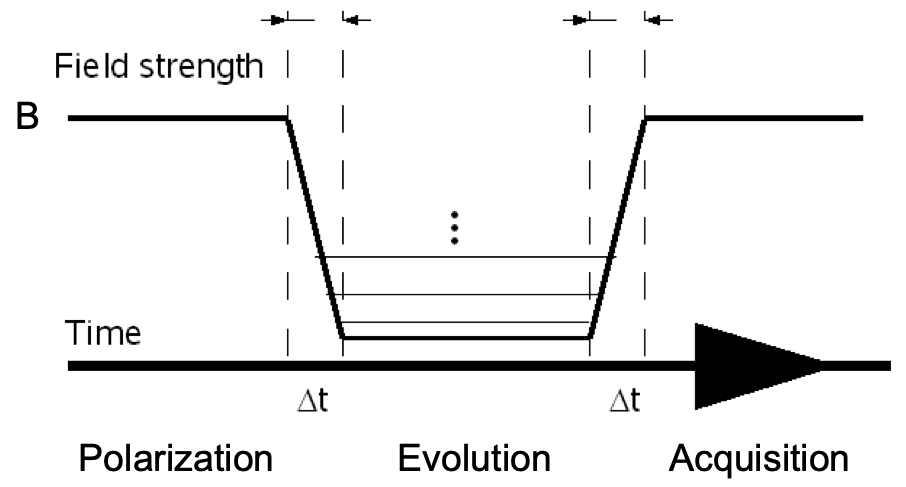
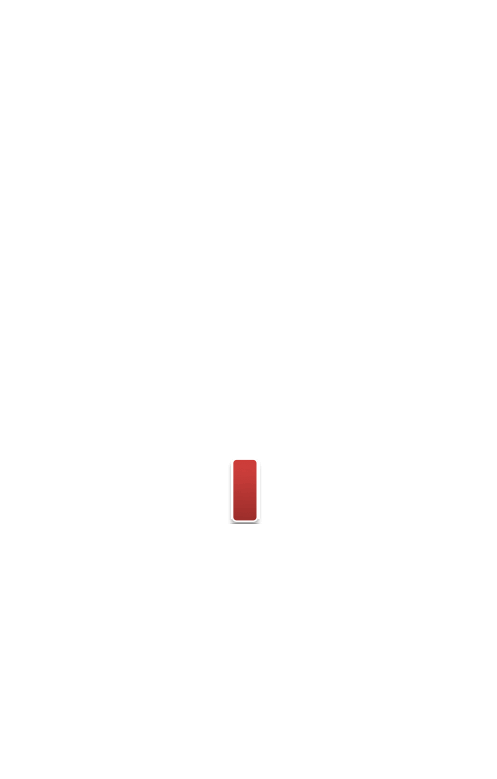
Welcome to this application note, specifically designed to guide researchers interested in the hardware development of sample shuttling in high-field Nuclear Magnetic Resonance (NMR). High-field NMR spectroscopy offers enhanced resolution, sensitivity, and wide applications such as chemical analysis, material characterization, and biomedical research.
Sample shuttling in high-field NMR involves precisely moving NMR samples within the NMR instrument using the stray field generated by the high-field NMR spectrometer. This technique enables dynamic experiments, including diffusion studies, relaxation measurements, and multidimensional spectroscopy, by manipulating the sample's position in the NMR spectrometer. Rapid and accurate NMR sample shuttling opens new opportunities for studying molecular dynamics, interactions, and structural elucidation.
- There are three key factors to consider when developing hardware for sample shuttling:
- Shuttling time:
Shuttling time refers to the duration comprising the travel time of the sample and the time required for it to equilibrate within the high-field magnet system. This time interval signifies the transition period from sample relaxation to data collection. To ensure accurate measurement of physical parameters, the measured time constant should exceed the shuttling time. While the shuttling time is significantly shorter than the measured parameters, one can achieve higher accuracy, as the magnitude will not decay during sample shuttling. - Stability:
Given the rapid movement of the sample, stability becomes a critical factor. Shuttling stability impacts the quality of spectra directly. For instance, while acquiring a two-dimensional NMR spectrum, an NMR sample must shuttle at least a hundred times within a single experiment. Each scan or serial data collection must occur under identical conditions to prevent the impacts on the data acquisition by the sample movements. - Compatibility:
The compatibility of the shuttle instrument is critical as it influences the preservation of the spectrometer's performance. The primary objective of sample shuttling in high-field NMR spectrometers is to collect field-dependent information while maintaining the performance of the high-field NMR spectrometers. Therefore, an optimal situation would be for the shuttle system to seamlessly integrate with commercial probeheads and NMR spectrometers without causing hardware impacts during sample shuttling.
Developing hardware for sample shuttling in high-field NMR spectrometers presents significant challenges. Limited space within the spectrometer's magnetic bore requires careful consideration to achieve high-speed and high-accuracy shuttling. The high magnetic field environment narrows down the material selection. Additionally, integrating commercial probeheads adds complexity due to narrow margins between the sample tube and probe head.
In the existing literature, many laboratories have employed this sample shuttling in high-field NMR to carry out field-dependent NMR measurements. They have developed various sample shuttling devices. Some utilize pneumatic power, allowing for rapid sample movement, although controlling air compression stability can be challenging. Others rely on motor control, which offers reliable and consistent actions but requires a precise mechanical design to achieve rapid shuttling while considering motor power, initial mass, and friction.
In the following table, we have compiled selected sample shuttle systems from the literature with their reported performance. The values presented in the table are extracted from the corresponding publications, ensuring transparency and accuracy in reporting the information.
Compatibility is a particular factor presenting the system's ability to adapt to different commercial probeheads. If a system is marked as "No" in the compatibility column, it indicates that it requires a specially designed custom-made probe head, limiting its compatibility with standard probeheads. It is important to note that the sensitivity and resolution of these systems depend on the installed spectrometer and employed probe head, so this table does not include sensitivity measurements.
Citation | Driving force | Shuttling time | Compatibility |
---|---|---|---|
S. Gross (1999) | Motor | 0.3s(tsh) in 62cm | No |
A. Redfield (2003) | Pneumatic | 0.1s(tsh) + 0.2~0.3s(teq) in 100cm | Yes |
H. Stork (2008) | Motor | 0.1s(tsh) in 24cm | No |
T-h. Huang (2011) | Motor | 0.1s(tsh) in 100cm | Yes |
A. Redfield (2012) | Motor | 0.25s(tsh) in 11T to 1T | Yes |
F. Ferrage (2013) | Pneumatic | 0.054s(tsh)+0.1s(teq) in 46cm | No |
T. Theis (2020) | Pneumatic | 0.5(tsh up)/1s(tsh down) + 1s(teq) in about 60cm | Yes |
F. Ellermann (2023) | Motor | 0.5s(tsh) + 0.4s(teq) in 30cm | adapted on tabletop NMR |
Note: tsh: sample shuttling time; teq: equilibrate time |
In conclusion, these various sample shuttle systems highlight notable advancements in hardware development for sample shuttling in high-field NMR. Among the discussed devices, T-h. Huang (2011) showed the shortest shuttling time and the highest compatibility. Their novel design of a mechanical field-cycling instrument achieves a comparable shuttling time to pneumatic control systems while demonstrating a shorter equilibration period. The system's successful adaptation to a commercial 5mm cryo-probe further validates its stability and sensitivity.
Other noteworthy systems include S. Gross (1999), who developed a sample shuttle system with an attached laser for field-dependent photo-CIDNP experiments. However, the limited availability of the custom-built probe head with an optical fiber attachment restricts its commercial application.
A. Redfield (2003) made significant contributions by introducing the first sample shuttle system for installation in commercial NMR spectrometers and probeheads. His later upgrade to a motor-based system in 2012 improved shuttling stability.
H. Stork (2008) presented a mechanical field-cycling device capable of shuttling samples from the center of the magnet dewar to the area beneath it. Although compatibility with different NMR spectrometers is limited, this system operates effectively across a wide temperature range of up to 1200K.
F. Ferrage (2013) and Bruker developed a sample shuttle device powered by air compression, employing a small section of an NMR tube. While A. Redfield's design (2003) relied on open control of air on top of the magnet, F. Ferrage's pneumatic control shuttle (2013) featured two air input/output openings, necessitating custom-made probe heads.
Additionally, T. Theis (2020) introduced an alternative sample shuttle system driven by air compression, utilizing high-pressure NMR tubes for enhanced performance.
Lastly, F. Ellerman (2023) developed a sample shuttle device driven by a motor, specifically adapted for benchtop NMR spectrometers. This device incorporates an additional tubing system for sample titration before sample shuttling.
These various systems contribute to the growing field of sample shuttling in high-field NMR, offering researchers a range of options to explore dynamic experiments, enhance data collection, and expand the frontiers of scientific discovery. While many of these systems have remained at the laboratory level, it is noteworthy that the sample shuttle system presented in T.-h. Huang (2011) has made significant strides in commercial development. Field Cycling Technology Ltd. has successfully brought this system to market and named it "High-Field Field-Cycler" (HFFC), exemplifying its practical applicability and widespread availability to researchers in the field.
References
Mechanical control shuttle devices
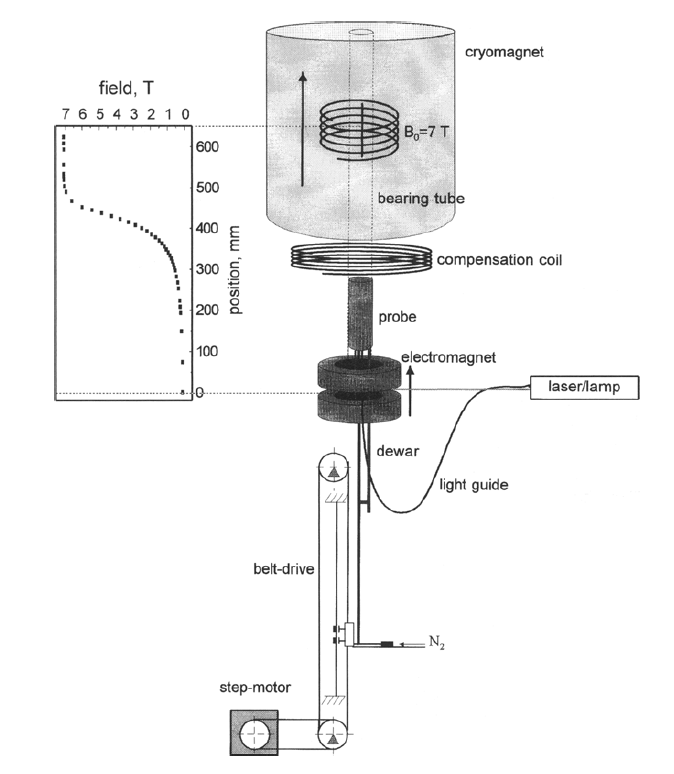
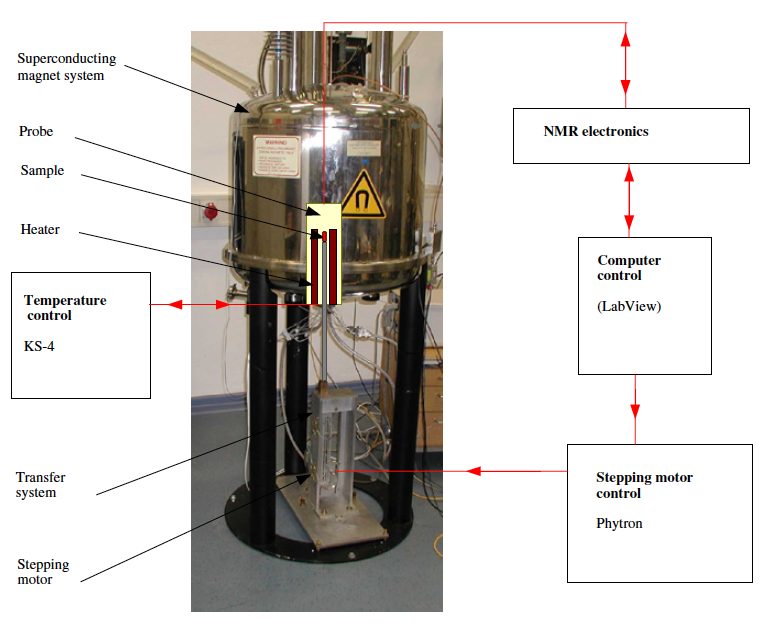
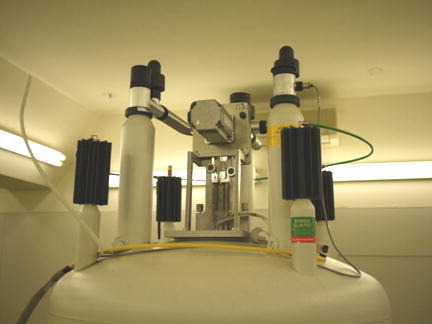
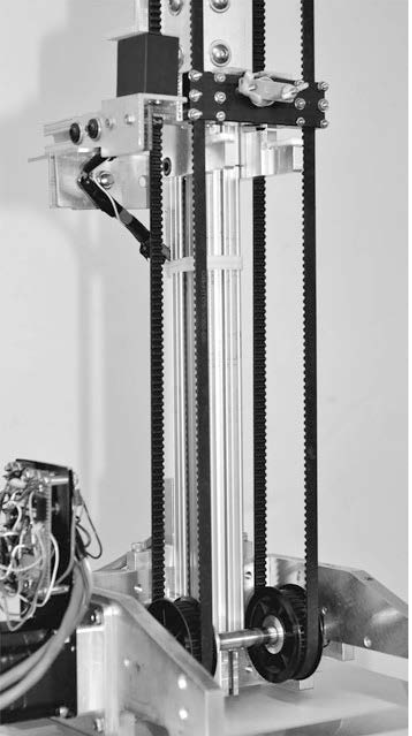
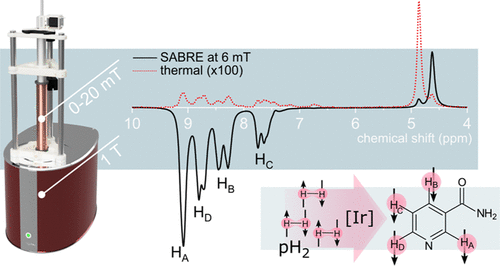